GLONASS in the age of hydrogen
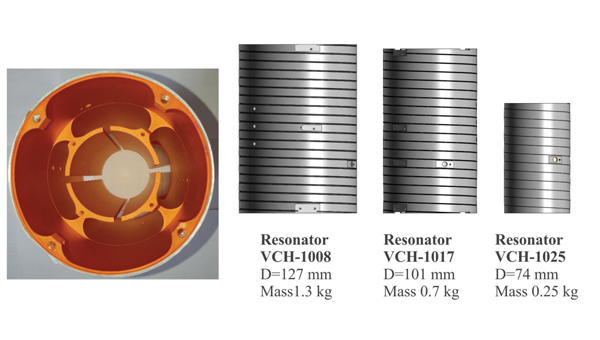
The development and digital transformation of the global economy are based today on high-precision time synchronization of transport management processes, the transmission of electricity and data and many other processes. The high-precision global synchronization of GNSS spacecraft signals makes global instantaneous all-weather navigation possible and is the primary solution to the problem of time transmission.
For the past 57 years, since their adoption by the International Bureau of Weights and Measures (BIPM) as a unit of time measurement in the International System of Units of the Atomic Second, the technical characteristics of atomic frequency standards have increased significantly. The universally used Coordinated Universal Time (UTC) is based on the atomic time scale (AT), the readings of which are adjusted taking into account data regarding the Earth’s rotation.
Since its inception, GLONASS has relied on basic solutions embedded in the definition of UTC(SU). AT is formed by calculating the total number of vibrations at the resonant frequency of the energy transition between the levels of the hyperfine structure of the ground state of the cesium atom (133Cs) in the absence of external influences. It was based on this substance that the first ground and airborne frequency standards for GLONASS were created.
In accordance with the system’s interface control document, GLONASS transmits Moscow Time1, which is established by law by the Russian Federation. GLONASS transmits UTC(SU), which is formed as UTC+3 hours. In recent years, much work has been done to modernize the infrastructure for the formation of the national UTC(SU) time scale2. Today, the State Standard of Time and Frequency (SSTF) of the Russian Federation is one of the most modern time synchronization centers in the world. The basis of the SSTF are hydrogen frequency and time standards (HS) of the active type. The composition of the SSTF includes a storage complex of the national time scale based on the six newest active-type HS with a daily frequency instability of 3×10-16. In total, the SSTF consists of 18 HS.
Including new HSs in the composition of the SSTF has led to a significant increase in its contribution to forming the UTC scale. Currently, 87 time standards at national time laboratories contribute to the formation of UTC, which, following the recommendations of the BIPM time department, regularly provides measurement information of standards of the established format and content. In turn, the BIPM time division forms the International Atomic Time Scale (TAI) and the UTC scale. On a monthly basis, the BIPM Time Department publishes the results of the international key comparisons CCTF-K001.UTC and other data based on which a comparative analysis of the metrological characteristics of national standards is carried out.
The ALGOS algorithm is used for the formation of TAI and UTC2. It includes two main procedures: forecasting the drift of the frequency of standards and determining the contribution of specific standards to the final result — determining the statistical weight of standards.
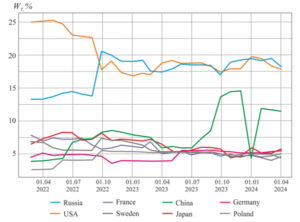
Figure 1: Dynamics of the contribution of the world’s major time laboratories. (All figures provided by the authors)
Starting in September 2011, forecasting of the standards’ frequencies takes into account the frequency drift inherent in hydrogen frequency standards. The procedure for determining the statistical weight of standards was also changed; preference was given to the predictability of their frequency, which showed a more balanced distribution of statistical weights and increased the contribution of hydrogen standards to the formation of TAI and UTC.
Figure 1 shows the dynamics of the contribution of the world’s main time laboratories to the formation of UTC for 2022-2024: Russia – the Main Metrological Center of the SSFT, SU; United States – Naval Observatory, USNO; China – National Time Service, NTSC; Japan – National Institute of Information and Communication, NICT; France – Paris Observatory, OP; Sweden – Technical Research Institute, SP; Germany – Institute of Physics and Technology, PTB; Poland – Time Laboratory, PL. The WT contribution of each laboratory is calculated as the sum of the weights of all frequency standards, the measurement results of which this laboratory transmits to the BIPM. The weight of each individual atomic time and frequency standard is calculated monthly by the BIPM time division when calculating TAI and UTC based on an assessment of its frequency stability over the billing period.
The main characteristic of the HS, which affects the characteristics of the formation of time scales, is the instability of the frequency of the output signals. To quantify the frequency instability of the output signals, a number of characteristics are used that reflect both random and systematic frequency changes over time. The Allan variation has been widely used for more than 50 years as the main assessment of the frequency instability of the output signals of standards in the time domain.
At the same time, in terms of frequency instability, the SSTF atomic clock also occupies a leading position. In October 2022, the average weight of atomic clocks of laboratories in the formation of TAI and UTC increased significantly, from 1 to 1.2% to 1.4 to 1.6% (the best indicator for foreign laboratories is 0.5 to 0.7%). This indicator is calculated by dividing the total contribution of each laboratory by the number of its atomic clocks that participated in the formation of TAI and UTC in the period under review. The average contribution more correctly characterizes the quality of the frequency standards of each time laboratory.
Additionally, an important indicator is the percentage of atomic laboratory clocks having the maximum allowable BIPM weight for an individual frequency standard. For 2022-2024, the figure ranged from 80% to 100%. The maximum possible weight depends on the total number of frequency standards involved in the formation of UTC in a particular billing period. This indicator was calculated as the number of atomic clocks having the maximum permissible weight divided by the total number of hours of this laboratory in each calculation period. All HSs from the SSFT have the highest rates of frequency instability and for most of the period under review make the maximum possible contribution to the formation of TAI and UTC.
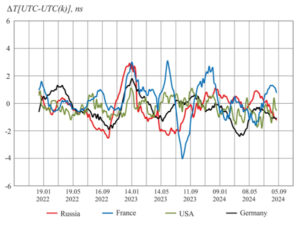
Figure 2: Comparative estimates of national time scale shifts. (All figures provided by the authors)
The characteristics of the Russian HS directly impact the formation of the national atomic and coordinated time scales of the Russian Federation TA(SU) and UTC(SU). Figure 2 shows the differences between national time scales and UTC.
Coordinated Universal Time(k) relative to UTC in 2022-2024.
The analysis of this data allows us to conclude that the UTC(SU) national coordinated time scale is one of the best national implementations of UTC, and the national atomic time scale TA(SU) occupies a leading position in terms of instability among the scales implemented in the leading national time laboratories.
GLONASS’ time scale is linked to the readings of the SSTF, taking into account the correction of the Moscow decree time and are implemented by the GLONASS synchronization system, which is also based on hydrogen frequency standards. Figure 3 shows the discrepancy between the values of the GLONASS system time relative to the UTC(SU) time, which is currently less than 10 ns.
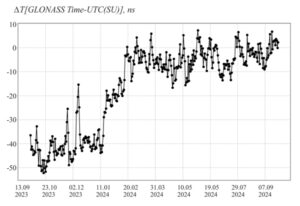
Figure 3: Error in transmitting the national time scale of the Russian Federation UTC(SU) using GLONASS. (All figures provided by the authors)
UTC(SU) parameters are transmitted via GLONASS spacecraft and the first of the fourth-generation spacecraft, which has a passive hydrogen maser (PHM) on board, was launched into operational orbit on Aug. 7, 2023. In the spring of this year, flight tests of this device began, which showed an improvement in the accuracy of time transmission during the transition from the cesium to the hydrogen standard.
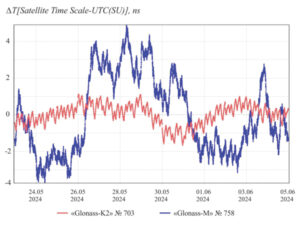
Figure 4: The discrepancy between the spacecraft time scale and the UTC scale: 1 – Glonass-K2, orbit slot 26, 2 – Glonass-M, orbit slot 12. (All figures provided by the authors)
Figure 4 shows the magnitude of the discrepancy between the time scales of the two GLONASS spacecraft. The cesium frequency standard installed on board the Glonass-M shows some of the best characteristics in the entire 20-year history of these spacecraft and demonstrates a daily relative frequency instability of 2×10-14, which is five times better than the design characteristics. Preliminary results of the use of the PHM as a source of reference vibrations and time synchronization pulses on board the Glonass-K2 in the same time interval shows a decrease of more than two times in the fluctuation error of forecasting the time scale with a relative daily instability of 7 × 10-15.
Similar characteristics emerge when using the most conservative spacecraft control scenario. Here, the update of the on-board clocks parameters (OCP) of the on-board time scale departure model relative to the system is carried out once per turn, i.e. once every 11 hours and 45 minutes. This ensures a decrease in the value of the OCP contribution to the error of navigation definitions due to the space complex before updating them from 1.4 m with a daily instability of 1×10-13 to 0.1 m for a relative daily instability of 7×10-15. The results obtained make it possible to redefine the basic onboard standard and switch to the priority use of the HPM signal for the formation of reference oscillations and clock synchronization of GLONASS spacecraft signals.
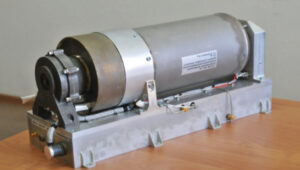
Figure 5 Passive hydrogen maser VC-1017. (All figures provided by the authors)
Further development of this technology provides for installing a VC-1017 small frequency standard on board (Figure 5). Compared with the one presented in a previous article, this standard is more than two times lighter while maintaining the stability characteristics of the formation of reference vibrations (Figure 6).
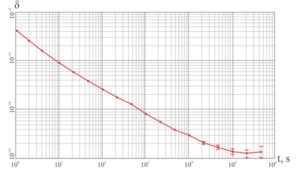
Figure 6 Measured instability of the VCH-1017 frequency relative to the state primary standard of time units, frequency and Russia’s national time scale for 40 days. The frequency drift was 1.51×10-15. The daily instability with the exception of drift is 1.42×1. (All figures provided by the authors)
The launch of the Glonass-K2 spacecraft with the prototype of this HPM is planned for the first half of 2025. Its precision characteristics allow us to expect a further increase in the stability of time transmission by GLONASS.
A significant improvement in the metrological characteristics of hydrogen frequency standards is achieved using a new system for forming a beam of atoms in a single energy state, which was developed before their industrial use. Thus, the daily frequency instability in active hydrogen frequency standards using a new beam formation system reached the level of (6-8) ×10-17.
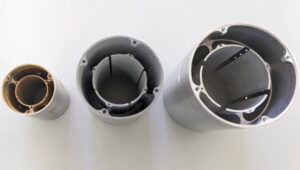
Figure 7: Microwave resonators for passive hydrogen frequency standards. (All figures provided by the authors)
The basis for creating passive frequency standards of various sizes was the pioneering invention of an oscillatory structure, which is used in all passive hydrogen frequency standards for global navigation satellite systems. This microwave resonator made it possible to change the dimensions at a constant resonant frequency of 1,420,405,751 Hz, close to the frequency of the atomic transition. At the same time, the design proved to be suitable for harsh operating conditions, including requirements for the launch of the spacecraft. (Figures 7 and 8).
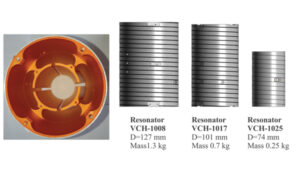
Figure 8: Microwave resonators of different sizes for passive hydrogen frequency standards. (All figures provided by the authors)
Reducing the size of the “heart” of the frequency standard allows you to reduce the weight of all other structural elements — thermostats, magnetic screens, vacuum caps, supports, etc. At the same time, it is easier to achieve structural rigidity requirements and reduce the influence of destabilizing factors.
The most important factor influencing the metrological characteristics of passive hydrogen frequency standards is the automatic frequency tuning system of the quartz oscillator to the frequency of the spectral line.
Careful engineering of the auto-tuning system avoids the influence of control circuits on each other. In addition, a technical solution has been introduced in the new frequency standards of the GLONASS system, which makes it possible to reduce the impact of various factors on the stability of the output frequency.
The results obtained allow us to consider the coming year 2025 as an important milestone in the development of time transmission technologies through the GLONASS system.

About the Author: Sergey Karutin
Sergey Karutin is the GLONASS designer general.
About the Author: Sergey Donchenko
Sergey Donchenko is general director of the Federal State Unitary Enterprise, Russian metrological institute of technical physics and radio engineering, VNIIFTRI FSUE.
Follow Us